By Dan Rubinstein
Photos by Luther Caverly and DEAP
In a cramped passageway that leads to the cavernous Cube Hall at SNOLAB, a world-class science facility deep below the surface of the planet at Vale’s Creighton nickel mine in Sudbury, Ont., Carleton Physics Prof. Mark Boulay climbs a stepladder to inspect a cooling system built around a 3,000-litre tank of liquid nitrogen.
The constant supply of cryogenic nitrogen is fed downward through a series of tubes and apertures to a two-inch-thick spherical acrylic vessel, where it keeps 3.6 tonnes of argon in liquid form at a temperature of about -180 Celsius.
That argon is the core of the DEAP-3600 experiment — an attempt to detect an invisible substance known as dark matter, which is thought to account for roughly one-quarter of the universe’s energy density. Dark matter is believed to outweigh normal matter (the atoms we are familiar with) by a factor of five to one, even though its existence has so far only been inferred by its gravitational effects on stars and galaxies and other indirect measurements.
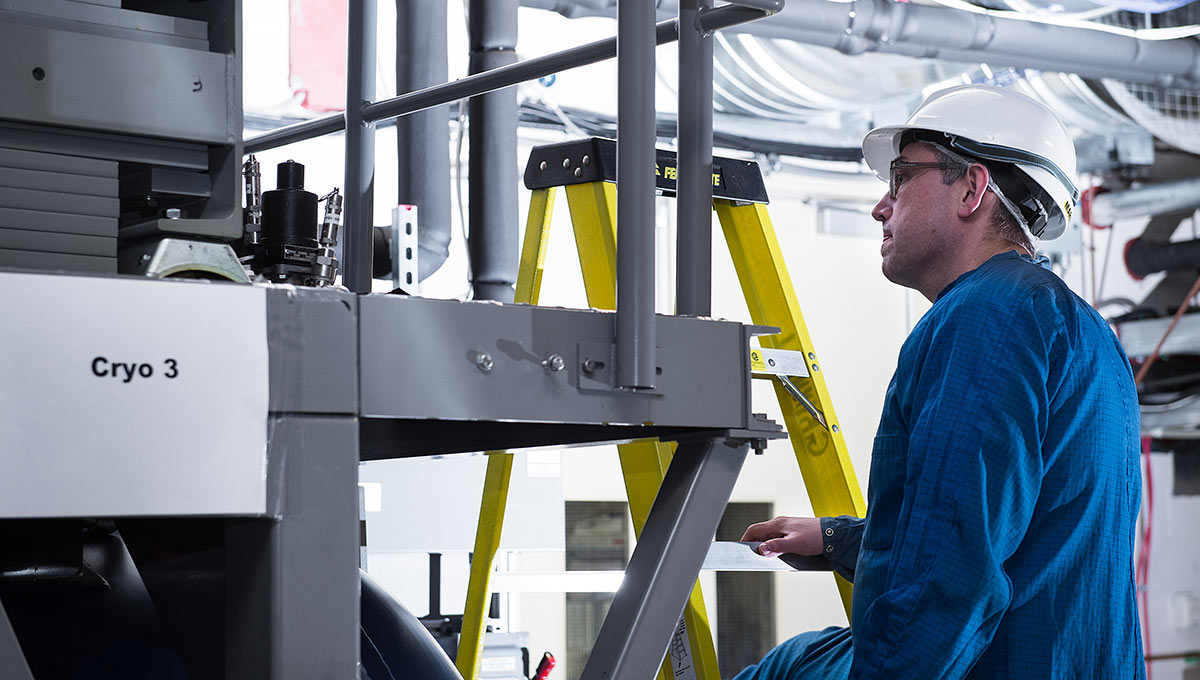
Carleton Physics Prof. Mark Boulay climbs a stepladder to inspect a cooling<br /> system built around a 3,000-litre tank of liquid nitrogen.
With the two kilometres of rock overhead blocking most of the cosmic radiation that reaches Earth, and with 10 times more sensitivity than any other comparable experiment, the DEAP detector could allow scientists to observe and identify dark matter by tracking the faint light pulses that result from elastic scattering of dark matter particles when they hit argon nuclei. But before they can solve a mystery that has been confounding physicists for decades, Boulay and his collaborators — a group of more than 75 researchers from 10 institutions in Canada, the United Kingdom and Mexico — must first address a seemingly endless string of details.
Today, up on that ladder, several months after the experiment was first fired up in November 2016, Boulay and SNOLAB Operations Supervisor Tony Flower are trying to figure out how to fix a bug in the cryogenic system. When it runs, as it is expected to do for years, it vibrates, and that movement appears to be damaging and loosening some of its components and fittings.
“This is typical of the lower-level mechanical problems we’re sorting out,” says Boulay, DEAP’s project director and a Canada Research Chair in Particle Astrophysics and Subatomic Physics.
“We’re certainly dealing with a lot of technical and engineering challenges, but the concept of a particle scattering off a nucleus like a billiard ball is fairly straightforward. At a fundamental level, what we’re doing is pretty simple, yet it’s one of the biggest questions in particle physics, and maybe in science.
“This is by far the dominant stuff in the universe,” he says about dark matter, “and we still have essentially no clue what it is.”
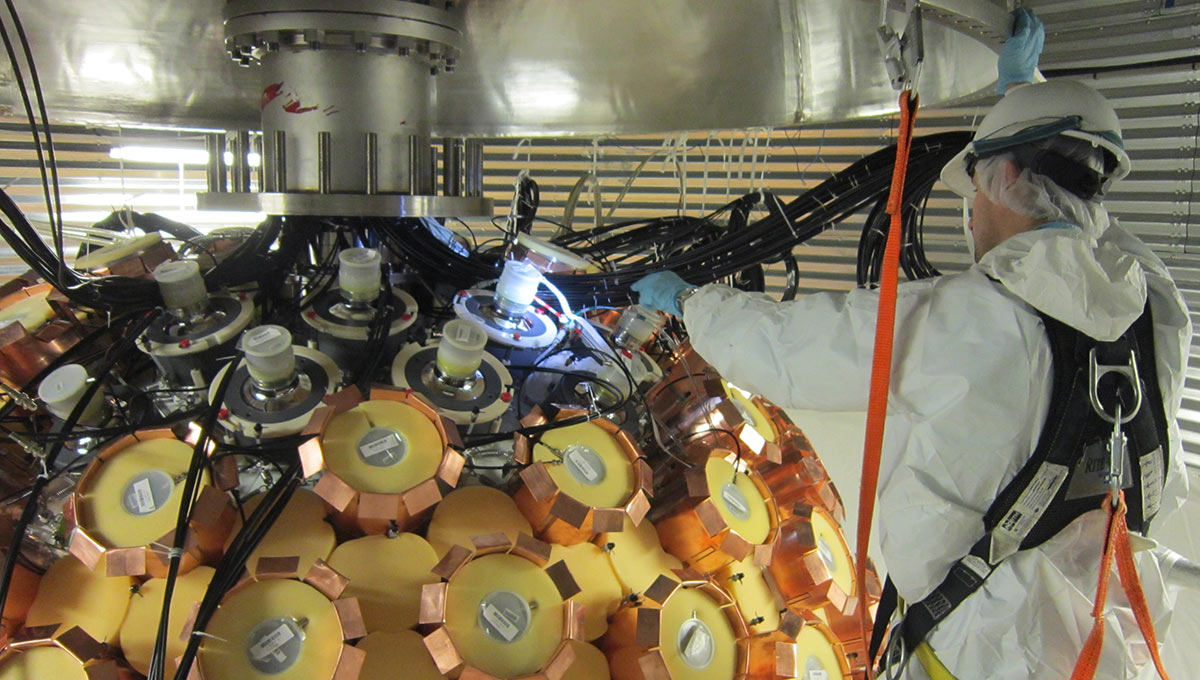
Zeroing in on the
Quest for Dark Matter
In a sense, Boulay was destined to lead DEAP. He was born and raised in Sturgeon Falls, an hour east of Sudbury on the Trans-Canada Highway, and became interested in physics at a young age. “The thing I liked about it,” he says, “is that you’re required to understand some fairly simple concepts but there’s very little memorization, which I was never very good at. So I always excelled in physics, because once you understand a few basic concepts you can go a long way.”
Boulay earned an undergraduate degree in physics at Laurentian University in Sudbury when the Sudbury Neutrino Observatory experiment was starting, exposing him to particle physics, and stayed at Laurentian for his master’s degree. He got involved in the final assembly of the SNO detector while working toward his PhD at Queen’s University, and had an opportunity to analyze its first results. Then he zeroed in on the quest for dark matter.
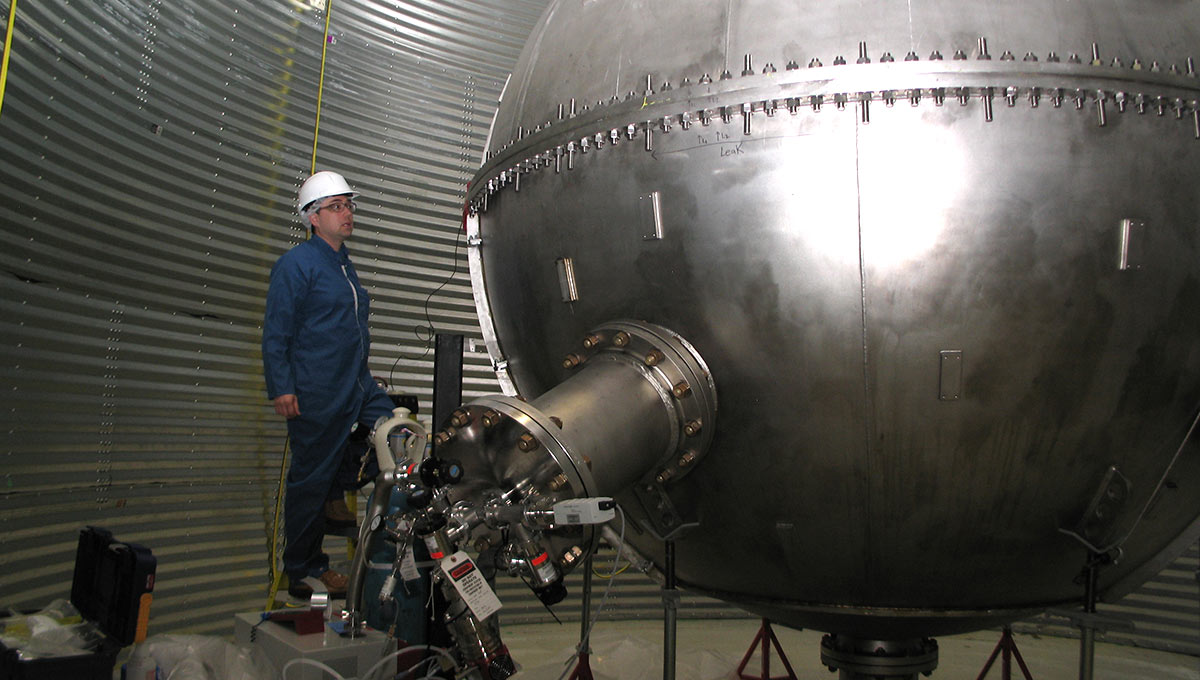
Boulay works inside the water tank during installation of the DEAP detector.
The term “dark matter” was coined in the 1930s by Swiss astronomer Fritz Zwicky, who, according to the DEAP website, “determined the mass and velocity distributions of objects within the Coma galaxy cluster and found that the velocity distributions he calculated implied the cluster had much more mass than the observable light suggested.”
Forty years later, American astronomer Vera Rubin corroborated the existence of dark matter by studying the rotation of galaxies and concluding they “rotated too fast for the gravitational force of their visible stars to hold them together.” Her results “pointed to the existence of vast amounts of unobservable mass, even 10 times more than visible, surrounding galaxies of stars.”
Several dozen experiments around the world are currently on the hunt for dark matter, including work at the Large Hadron Collider near Geneva, the LUX underground experiment in South Dakota, the XMASS project at Japan’s Kamioka Observatory, and the IceCube Neutrino Observatory, a particle detector at the South Pole.
But DEAP, which was conceived around 2004, when Boulay was doing post-doctoral studies at the Los Alamos National Laboratory in New Mexico, represents perhaps the best shot scientists have. Its potential is rooted in the unique properties of argon, which will help researchers discriminate backgrounds when they study the recoil of scattering particles, as well as the large volume of argon being deployed. Every other component of the detector has an extremely low level of radioactivity, which will allow the experiment to capitalize on the sensitivity of its location deep underground at SNOLAB.
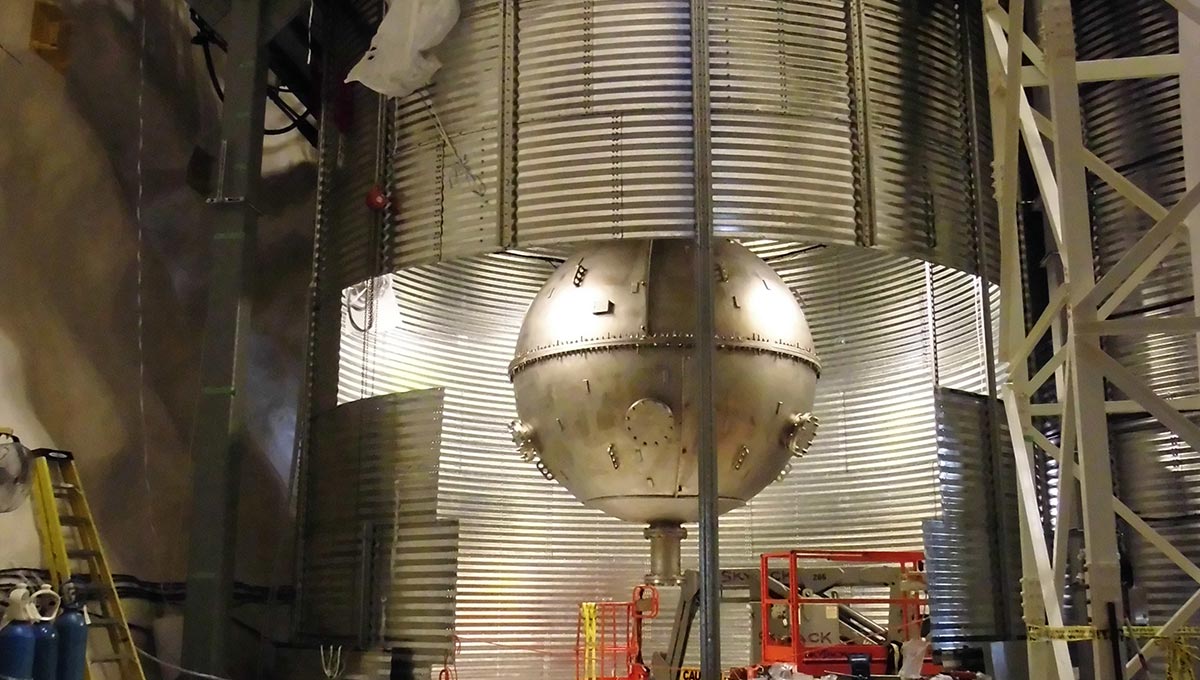
Scaling up the DEAP Detector
In 2006, Boulay led the development of a small prototype detector with liquid argon at Queen’s to demonstrate that this process could work. That design, and expertise from the SNO experiment, informed the construction of the full-scale version.
The argon vessel, which has an inner radius of 85 centimetres, is surrounded by 255 photomultiplier tubes (PMTs) to capture the light pulses, and the whole apparatus is immersed in a water tank to further block external radiation. Since it was turned on last November, much of the focus at DEAP has been calibrating the detector and collecting preliminary dark matter search data in anticipation of collecting up to 10 to 15 dark matter scattering events during the full four-year detector running period.
When the detector is triggered by a radioactive event, data is recorded from each of the PMTs at a rate of about five megabytes of data per second. That will add up to roughly 250 terabytes per year. All this information is stored (and backed up) at a data centre that’s part of the Compute Canada network and is analyzed by researchers from the project’s partner institutions.
The DEAP team released the experiment’s first results at a conference in Sudbury in late July, a paper that demonstrated the best-ever rejection of radioactive backgrounds using liquid argon, and the lowest level ever achieved of background radon, one of the most troublesome backgrounds in dark matter searches.
“The early indications are positive,” Boulay says about the data analysis conducted to date. “We know that dark matter exists and affects our universe at many scales. The hypothesis that many people have is that it’s a new particle that we’ll be able to detect directly. What we don’t know is that mass of that particle, or how often it will interact with regular matter. When you mount an experiment like this, those two parameters are unknowns, and if you observe something you can pinpoint which sets of those parameters — dark matter particle mass and its probability of interacting — are consistent with the observation. If you don’t see anything, you can rule out part of that parameter space and push farther and farther down in sensitivity.
“It’s extremely exciting be part of this experiment,” he continues. “It’s exciting to have the world’s leading facility for underground science so easily accessible to us. At any point in the next six months or year, we may for the first time see direct evidence of this material that essentially the universe is made of. It would certainly be a paradigm shift. Once you have direct observation of a dark matter particle, you can start looking in more detail at the data and rule out or rule in various theories of particle physics. It will provide a road map for where to go in particle physics.”
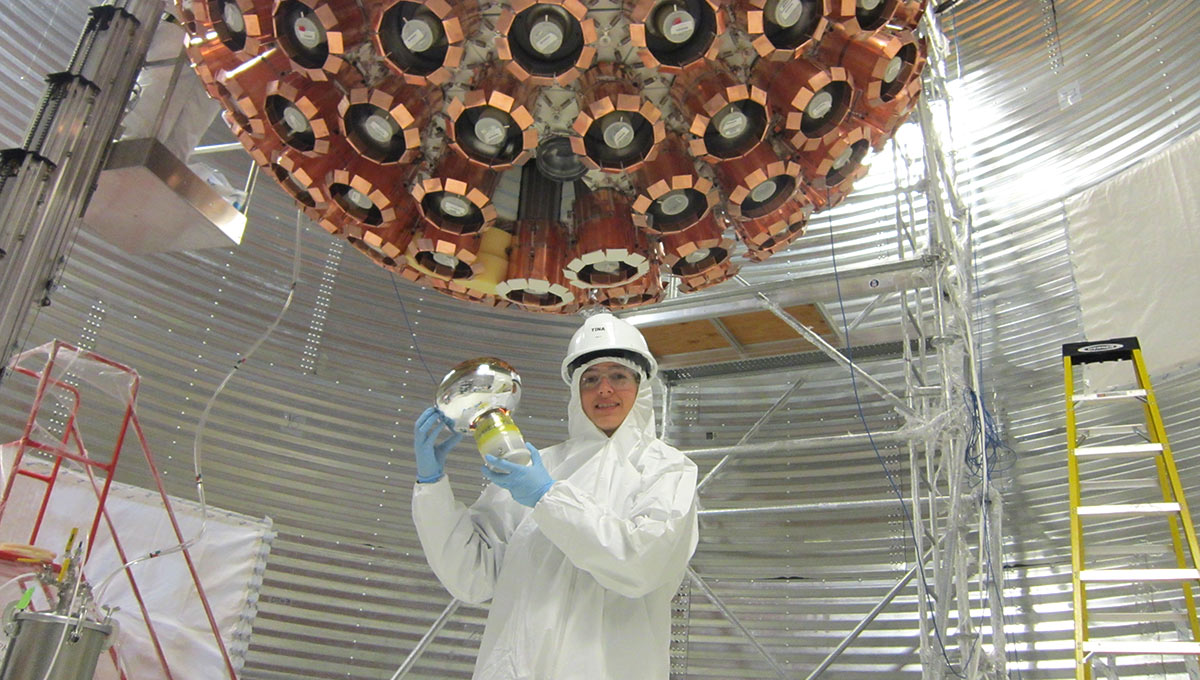
A Natural Evolution
After getting his training and starting his research career on the SNO experiment, DEAP is a natural evolution for Boulay. There’s pressure to maintain the high standards that were set with SNO, he says. In a sense, that foundational work would be considered low-hanging fruit today — “they knew what they were looking for” — whereas DEAP is more of a voyage into the unknown.
“With SNO, we knew one way or the other, as long as the experiment could be made to work technically, that the answer would be extremely interesting,” says Boulay. “Most experiments right now in particle astrophysics are probing the unknown. In the case of SNO, we knew we would either see that neutrinos changed flavour or they didn’t — and either one of those would have been a momentous result. Now we’re searching for things with experiments such as DEAP and nEXO and there’s not really a firm prediction about what we will see. So, we’re more in a search mode, trying to discover the unknown.
“The way I would phrase it: we’re opening a new window on the universe that we haven’t looked through before.”
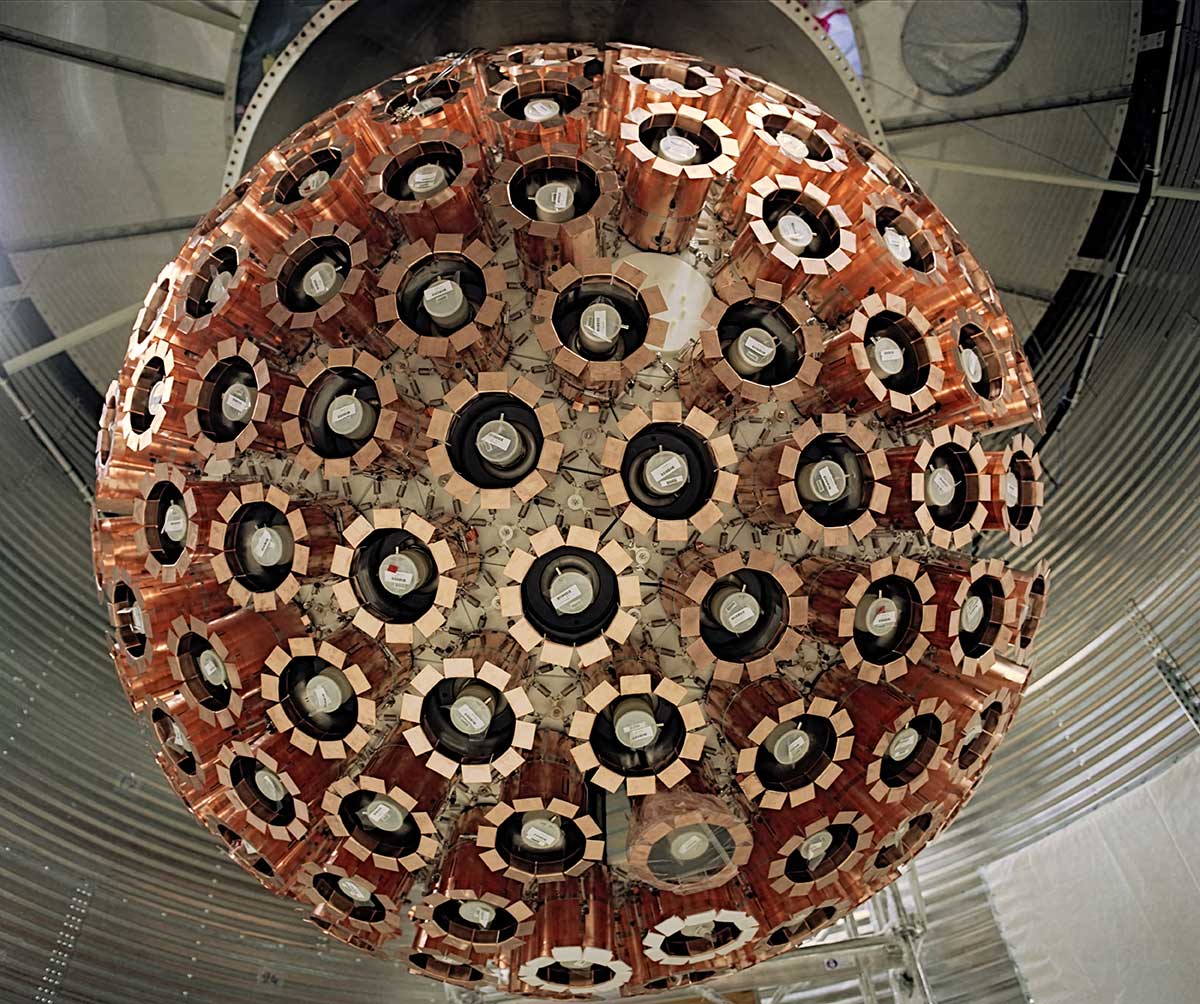
Friday, August 25, 2017 in Faculty of Science, Innovation, Research
Share: Twitter, Facebook