By Tyrone Burke
Photos by Fangliang Xu
Even if we can’t see them, they are always there.
Neutrinos are among the most abundant particles in the universe, but they are many thousands of times smaller than an atom. They have almost no mass, no electrical charge, and travel at the speed of light. These tiny, subatomic particles are created by radioactive decay – in the nuclear fusion reactions that power our sun, or nuclear energy on Earth.
When distant stars explode in a supernova, they send neutrinos hurtling through space at near light speed. For billions of years, neutrinos can travel uninterrupted across the universe. Even planets are no obstacle. Since neutrinos hardly interact with matter, they pass seamlessly through rocks, metals and other materials. There are even neutrinos passing through you right now.
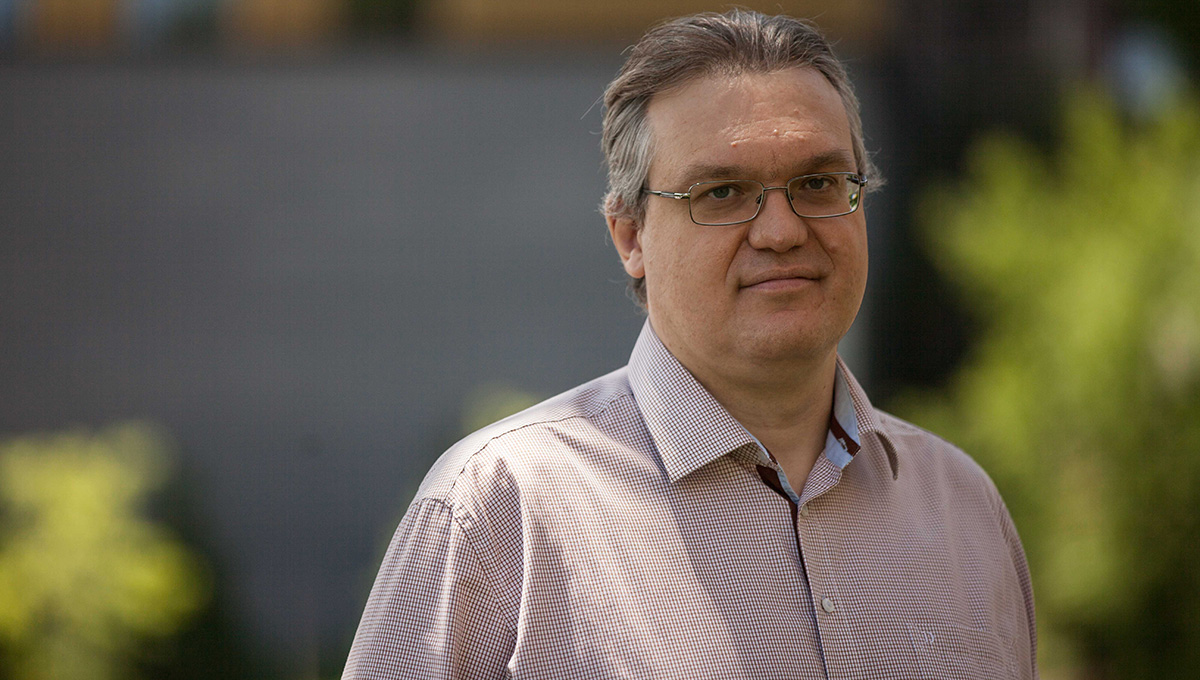
Prof. Razvan Gornea
“On Earth’s surface, we are constantly bombarded by radiation, and we have evolved to live with this,” says Razvan Gornea, an experimental physicist and assistant professor in Carleton’s Department of Physics.
“There is radiation from the sun, and high energy particles coming from outside the solar system. Those are called cosmic rays and are produced by many astrophysical sources in the universe.”
When high-energy cosmic rays interact with Earth’s atmosphere, they create an atmospheric shower of charged particles called muons and pions. The muons are able to reach Earth’s surface, and their presence makes it difficult to detect neutrinos at ground level. To do that, you need to go underground.
“The deeper you go, the more high-energy particles are removed,” Gornea says.
“By going five or 10 metres underground, you can remove some of the particles that interfere with our very sensitive detectors, but muons can penetrate much deeper. On rare occasions, the highest energy muons reach the Sudbury Neutrino Observatory (SNOLAB), which is two kilometres underground.”
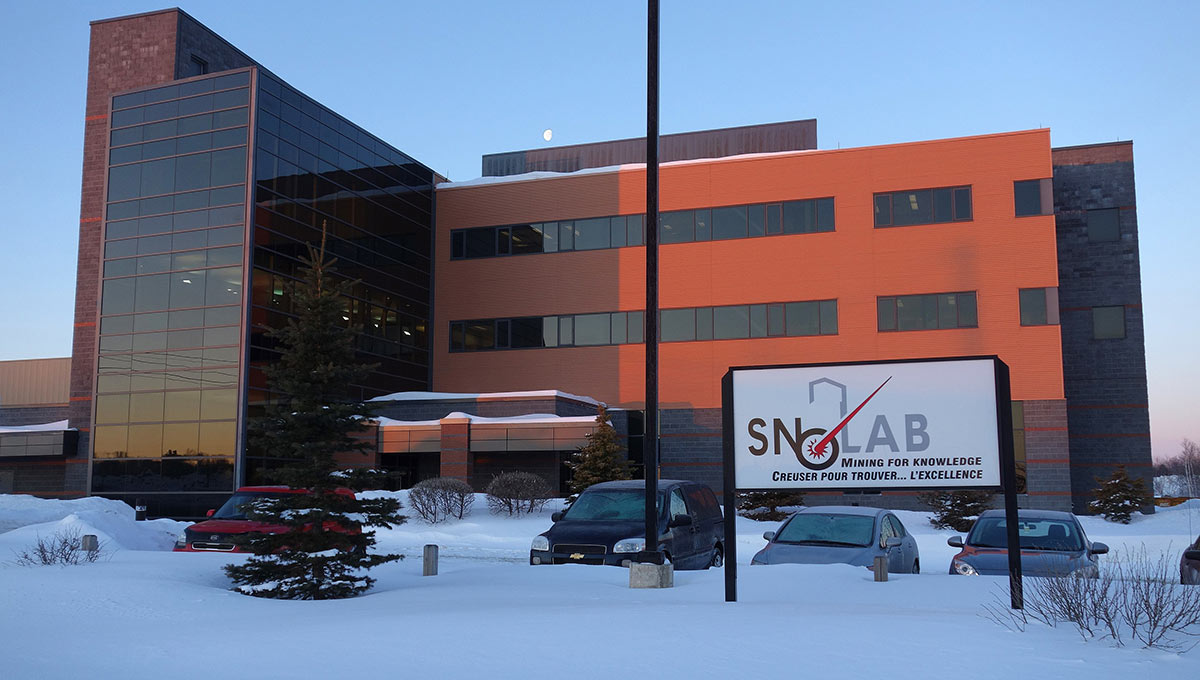
The SNOLAB facility, located at an active nickel mine in Sudbury, Ont.
To detect neutrinos, physicists use Cherenkov radiation detectors. These tanks of ultra-pure water are buried deep below Earth’s surface, and lined with light-sensitive devices that can identify miniscule amounts of light. Neutrinos only rarely interact with matter, but occasionally, they do interact with the hydrogen or oxygen atoms in water. With trillions of neutrinos passing through a Cherenkov detector every second, it is these rare interactions that physicists are trying to find.
When light travels through water, it travels more slowly than it does in air. And when neutrinos interact with hydrogen or oxygen, this produces an electrically charged particle that travels at nearly the speed of light – faster than light itself can travel in the water of the Cherenkov detector. As long as the particle travels faster than light, it will create a dim cone of light – an effect similar to the shock waves in the atmosphere when a supersonic jet breaks the sound barrier.
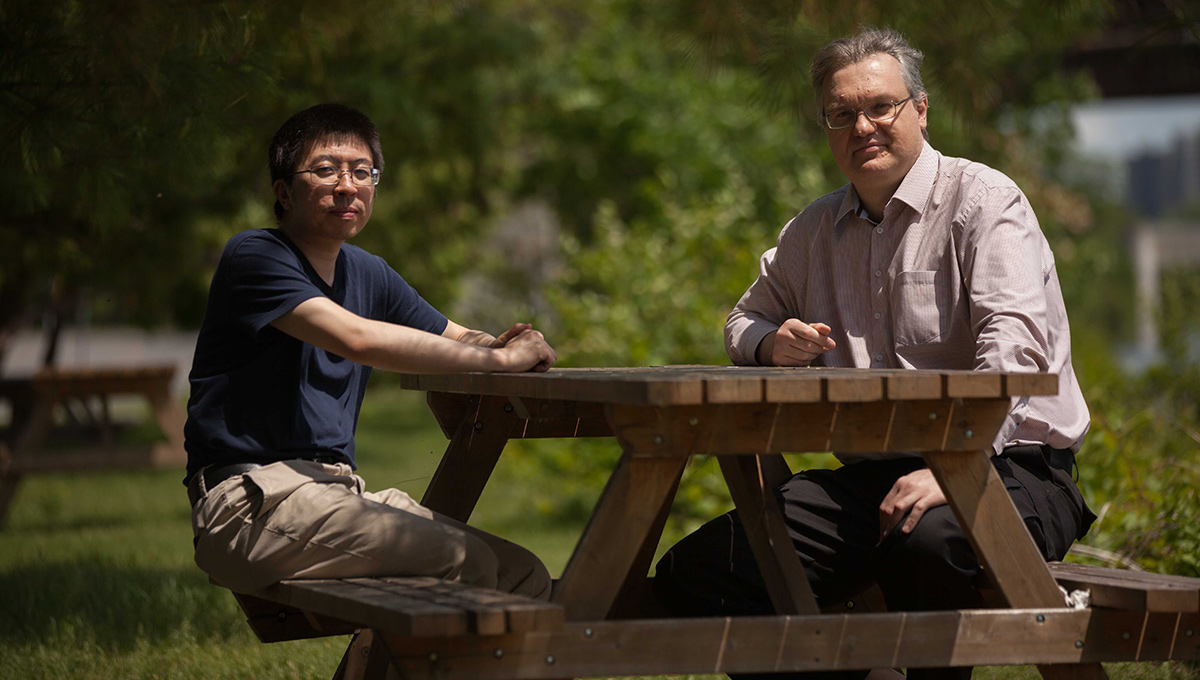
New Light Detection Technology Could Lead to Better Measurement
In Japan’s Gifu Prefecture, 1,000 metres below the surface of Mount Ikeno, construction is set to begin on an enormous Cherenkov radiation detector. Hyper-Kamiokande – Hyper-K for short – will consist of a cylindrical tank 68 metres in diameter and 71 metres tall – or about the height of a 21-storey building. It will hold more than 250 million litres of water, and its walls will be lined with 40,000 light-sensitive devices called photomultipliers.
Hyper-K’s size will greatly increase the chances of observing neutrinos, but it also creates challenges. The type of photomultiplier currently in use has relatively low resolution, and Gornea believes that higher resolution photomultipliers could make Hyper-K even more valuable.
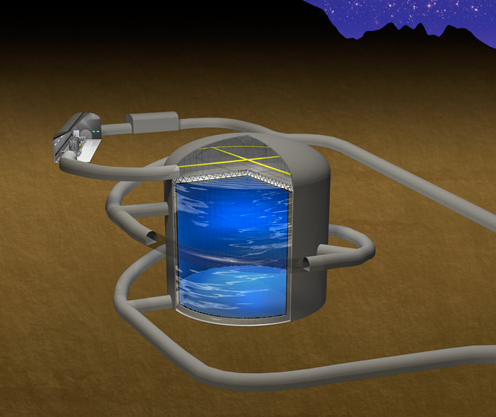
Hyper-Kamiokande
“Each photomultiplier is like a large tube – 50 centimetres in diameter,” says Gornea.
“This is the size of each ‘pixel,’ and the resolution is low. In a way, you have to reconstruct the faint rings of light with pixels that are 50 centimetres each. You can do lots of particle identification and energy reconstruction with this, but the Canadian team is proposing a sensor which has the same diameter, but has higher granularity. Inside, we propose to install 19 three-inch photo multipliers. This would have higher definition to detect wrinkles of light.”
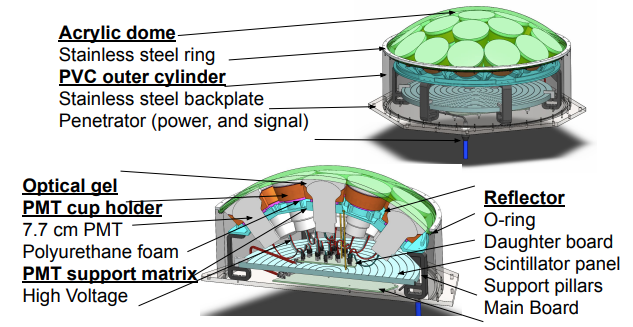
multipurpose photomultiplier concept (mPMT)
This multipurpose photomultiplier concept (mPMT) is in the design and testing stage, and a team of researchers from Canadian universities is working to demonstrate its benefits. Its higher granularity could more accurately measure known phenomena like neutrino oscillations, and expand the range of topics Hyper-K can explore.
This could include phenomena beyond the Standard Model of particle physics – like proton decay, or yet-unknown subatomic particles. One such particle is proposed by Yue Zhang, an assistant professor in the Department of Physics.
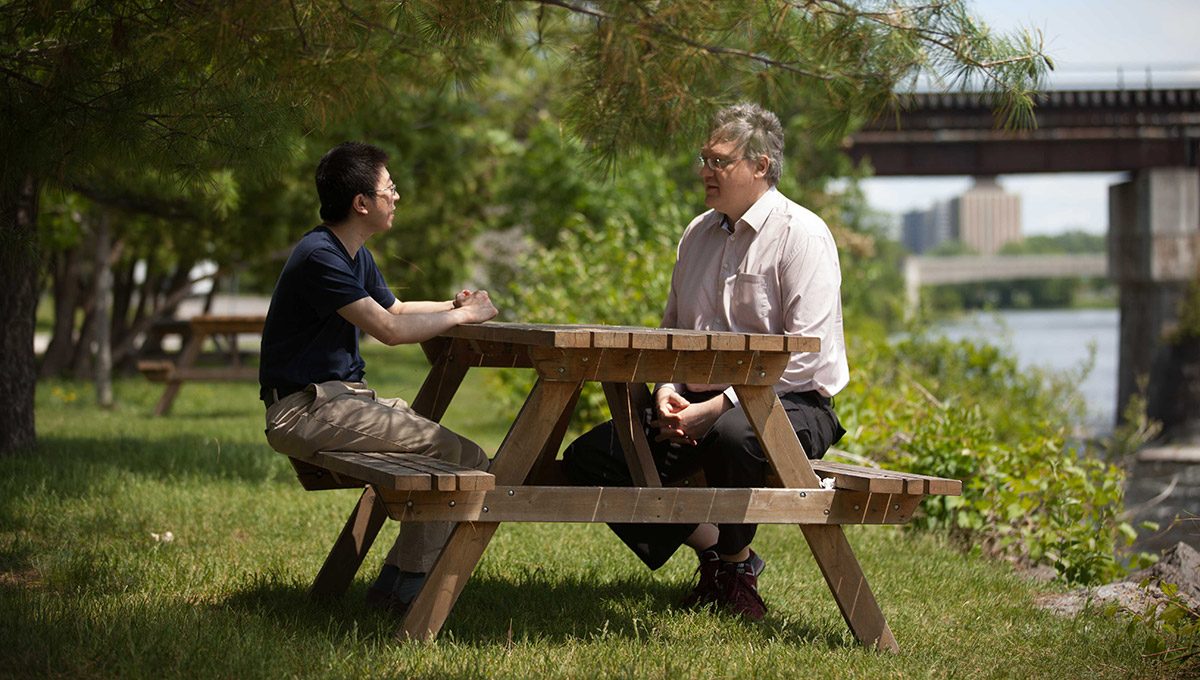
Carleton Theoretical Physicist Proposes Particle Beyond Standard Model
“I’m looking for something that we don’t know yet whether it exists or not,” says Zhang.
“Dark matter is a mystery of nature, and we want to understand what it is. In one class of model, dark matter interacts with us through a new force-carrier – a type of intermediate particle that enables the transfer of energy between particles. This particle would mix with the Higgs boson particle that was identified in 2012, using the Large Hadron Collider at the European Organization for Nuclear Research (CERN).”
Zhang predicts that the interaction between high-energy cosmic rays and Earth’s atmosphere would produce an extremely light force-carrier particle that would travel through the Earth. The particle would have a neutral charge, but would decay into a pair of particles. One would be an electron and the other a positron, conserving the neutral electric charge of the original particle.
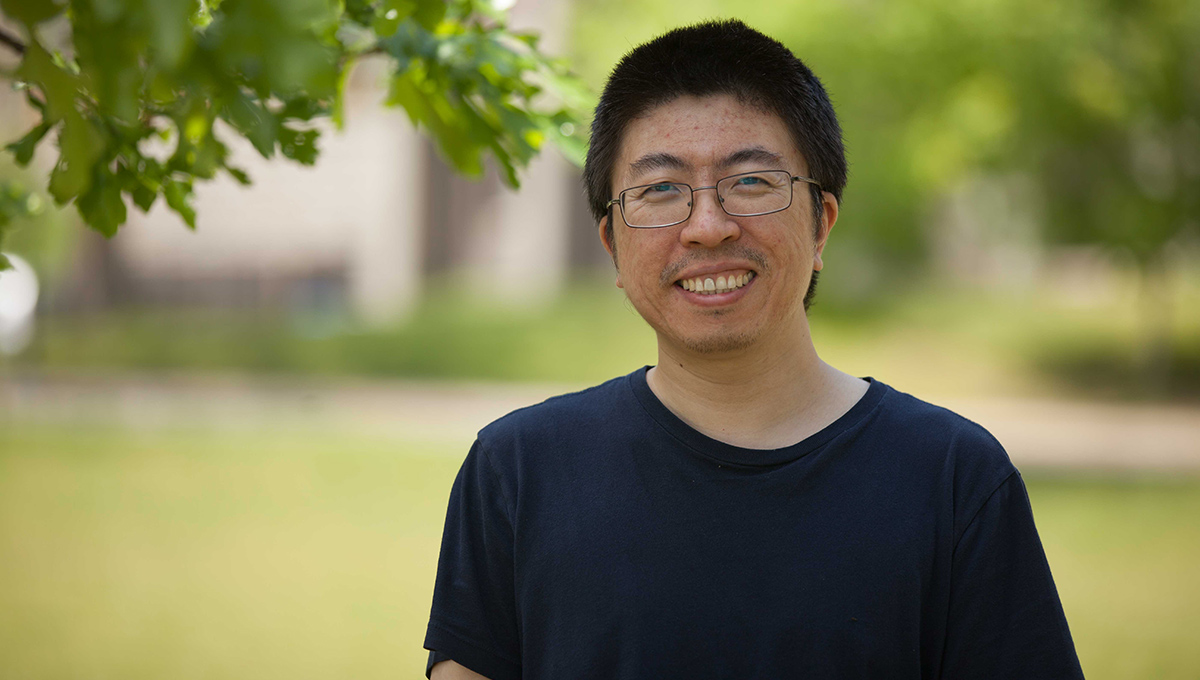
Prof. Yue Zhang
It could be identified by a distinctive double-ring Cherenkov radiation signature, but the double-ring would be so small and faint, that highly sensitive light detection equipment like the proposed mPMT will be necessary to observe it.
Even then, it would need to happen in the right place, at the right time.
“We are looking for something that could happen anywhere, so we need to cast a wide net,” says Zhang.
“Hyper-K is special because it’s the largest detector that will be built anywhere in the world, in the near future.”
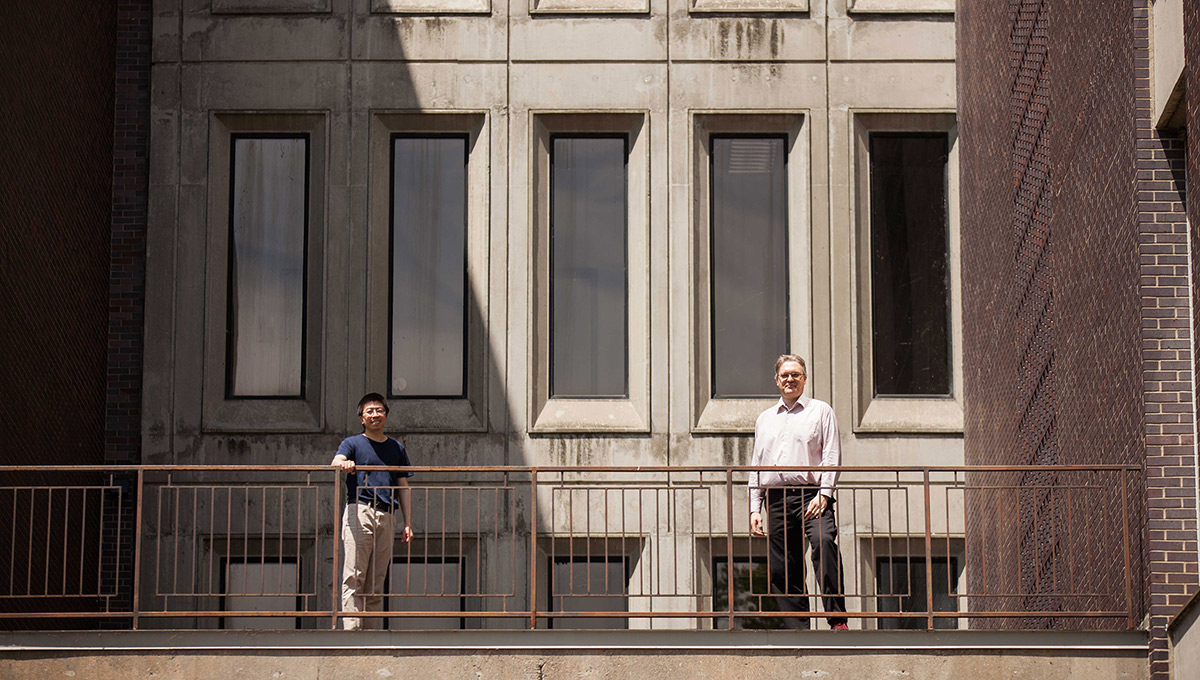